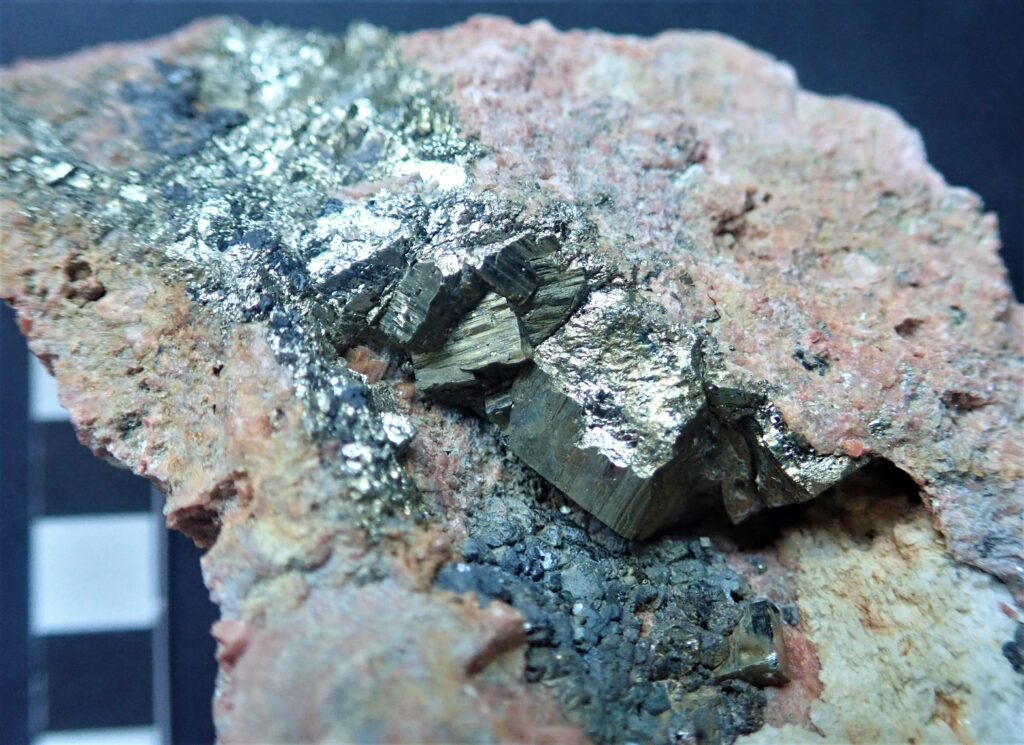
The assessment of ground aggressivity and suitability of construction materials are fundamental aspects of geotechnics. Ground hosted sulfide and sulfate minerals are known to produce unwelcome implications for ground engineering. Confidence in selection of the most appropriate form of construction and mitigation methodology, must be based on the results of meaningful site-specific material characterisation and assessment of performance in the likely construction environment. It is apparent that many ground engineering practitioners do not fully appreciate that certain rocks and soils are liable to contain sulfur species that may negatively impact ground engineering projects. In practice assessment procedures are often followed without a clear understanding of the issues and how to best manage them. This approach is not always suitable for managing the extensive variability encountered in the UK. Furthermore, an appreciation of how the construction activities may bring about undesirable changes are necessary for design of appropriate mitigation and long-term management. This short article discusses some of the issues that may occur, particularly where pyrite is concerned and considers how these characteristics and associated risks may be managed.
Causes of construction groundwork damage brought about through physical deterioration of ground and engineering materials are attributed to a wide range of factors that involve physical, chemical, and biological processes. In the UK a high proportion of such occurrences in the engineering environment result from the presence of sulfate ions in groundwater, brought about through dissolution or reaction of sulfur compounds. Pyrite and gypsum are the most commonly occurring sulfur compounds likely to be encountered during construction works. In certain locations, the source of the sulfate ions is clearly through dissolution of evaporitic deposits, but in many cases, covering a much wider geographical area, these are derived from the oxidation of iron sulfide minerals, particularly pyrite. Pyrite, and the other infrequent iron sulfide minerals are unstable in oxidising and damp atmospheric conditions typical of the construction environment and will rapidly weather, producing insoluble orange-brown hydrous iron oxide, with release of hydrogen (H+) and sulfate (SO42-) ions into solution as mobile sulfuric acid. This has a significant negative impact for ground engineering when reaction occurs consequent of ground disturbance, creating conditions that are aggressive to ground material including buried steel and concrete and, in some cases, raising sulfate to harmful levels.
Reaction of the sulfuric acid with other calcareous minerals such as calcite or concrete, give rise to selenite-gypsum as discrete crystals, and this involves expansion. The replacement of pyrite and calcite by selenite involves a volume increase of around 103%, developing ground stresses and causing differential heave due to indiscriminate crystal growth. This chemical alteration is frequently accompanied by rapid deterioration in engineering properties of the host material and the volumetric gain often causes disturbance in filled ground, and failures of foundations, earthworks, underground excavations, tunnels, and slopes. Observations have also documented abiotic pyrite oxidation where the pH of pore fluids was around pH >12, indicating that this reaction mechanism can also occur when pyrite bearing ground is treated using lime and cement.
The oxidation of pyrite is complex, it occurs through various reaction stages, at different rates, which conclude in a range of products. Ultimately reaction depends on various aspects including the crystalline form and grain size of the pyrite, the mineralogy and fabric of the host, and environmental conditions, including the exposure to weathering brought about by the engineering work. To manage any negative impacts to design and construction, the possibility of changes promoting the potential for pyrite oxidation during and after construction needs to be considered.
Sulfur is an abundant element in the Earth’s crust and occurs in geological materials of all ages and origins, in a variety of forms. Sulfur is highly reactive and readily combines with most non-noble elements, particularly under reducing conditions, to form metallic sulfides of which the iron form, pyrite (FeS2) is the most widely occurring, along with its less common dimorph marcasite (FeS2), and occasional pyrrhotite (Fe1-xS where x = 0 – 0.2). Gypsum tends to be the most widely occurring sulfate mineral and is frequently encountered during ground works. Gypsum occurs as a primary accumulation in evaporite deposits such as the Mercia Mudstone Group and forms through evaporation of saline waterbodies. But it is more widely occurring as the crystalline ‘selenite’ form, which tends to develop as a ‘secondary’ product of contemporary weathering action on pyrite in the presence of calcite. The oxidation of pyrite will also give rise to high concentrations of sulfate ions which are mobilised by groundwater. Not all forms of sulfur are troublesome in engineering situations, although this depends upon the environmental conditions. Some recalcitrant mineral sulfates, such as barytes, celestine, and organic sulfur are relatively stable in weathering environments, and do not contribute to the sulfur present in groundwaters, unless conditions are unusual, so they are unlikely to impact significantly in construction and geo-material applications. Therefore, knowledge of the likely occurrence and attributes may help to manage potentially adverse conditions that could occur during and after construction.
Pyrite is remarkably widespread in its occurrence and is found as a minor constituent in a wide range of naturally occurring materials. It occurs in rocks and engineering soils, ranging from ancient sediments to Recent deposits, igneous and metamorphic rocks and hydrothermally deposited mineral veins. Pyrite occurs as diverse forms including variously shaped grains, nodules, and well-formed crystals, ranging from microscopic to several cm across; the morphology of pyrite has an influence on its potential for atmospheric oxidation. Therefore, its appraisal may help to determine its potential reactivity and the suitability of pyrite bearing ground and geomaterials for particular applications.
The different forms of pyrite and their combinations all share the same internal arrangement of iron and sulfur atoms but conditions during formation affect the crystal form. Well-crystallised pyrite occurs in the brass-yellow macroscopic form as large masses, veins or as large discrete often striated cubic, octahedral or pyritohedral crystals a few millimetres to a few centimetres in dimension and are commonly referred to as the ’non-reactive’ form of pyrite. Typically, these are found in rocks that are well indurated and / or have been subjected to moderate to high-temperatures and pressures. These well-crystallised forms of pyrite have a densely packed structure and relatively small specific surface area such that they tend to respond slowly in weathering environments. Macroscopic forms of pyrite occur extensively in igneous and metamorphic rocks and some hard-rock limestones, sometimes in substantial concentrations distributed through the host and tend to be relatively stable in construction environments. These deposits are widely worked in the UK for construction aggregates in which slow oxidation or combination with cementitious binders may lead to problematic chemical reactions. In less-well indurated sedimentary rocks, pyrite may occur as visible nodules or smooth faced crystals, but more typically, it takes the form of disseminated microscopic framboids that are very difficult to recognise.
The microscopic framboidal form of pyrite is of greatest concern to ground engineering. Framboidal pyrite tends to form in sedimentary environments under anoxic reducing conditions through microbial activity where it remains stable, but when exposed to oxidising and damp atmospheric conditions it may rapidly deteriorate with consequentially detrimental effects. It is commonly found in dark coloured (grey and dark grey), fine-grained sedimentary deposits including clays, mudstones, argillaceous limestones, siltstones, sandstones, and low-temperature hydrothermal deposits. The microscopic reactive forms of pyrite may also occur in newly formed sediments, including marine sands and gravels and river flood plain deposits, which are widely used as construction aggregates. Framboids are raspberry-like spherules, typically 2 – 80 μm diameter, comprising of ordered agglomerations of microcrystalline pyrite grains that are themselves <0.3 – 2 μm in diameter. They occur as disseminated spherules, clusters, or dark greenish-grey coloured concentrations along partings. The framboidal structure results in grains with a large surface area in proportion to their volume, making them highly susceptible to oxidation in an oxygen and water bearing atmosphere and oxygenated water. This reaction may be mediated and greatly accelerated by microbial intervention from bacteria such as the ubiquitous Acidithiobacillus sp., which rely on electron transfer between Fe2+/Fe3+ for their metabolic process and this functions as a key mechanism in the oxidation reaction. In the ground engineering discipline, this form of pyrite is often referred to as the ‘reactive’ form of pyrite.
It is cautioned that the allusion to the visible form of pyrite as ‘non-reactive’ is not strictly true, the well-crystalline macroscopic forms of pyrite are still susceptible to oxidation following exposure, but depending on their surface condition, reaction generally will occur over a much slower timescale and may not be considered significant where construction is concerned, although it may be expedited where physical damage occurs to the crystals and through reaction of less stable forms. Therefore, potential reactivity must be assessed, with judgement also relying on previous experience of that material.
In the UK, framboidal pyrite is widely found in the dark coloured deposits of marine and fluvial origins of Carboniferous, Jurassic, Cretaceous and Eocene age. These account for a large part of the near-surface stratigraphy that contains many major urban centres. Through weathering, sulfate minerals can be present at shallow depth, whereas sulfide minerals may predominate at greater depths where oxidation has not occurred. Weathering involves physical and chemical changes to the natural material as it adjusts to different overburden pressures and the presence of atmospheric gases. The change in pressure results in development of fissures and joints which facilitate the movement of oxygenated groundwaters. Water movement promotes chemical adjustments including hydration, dissolution and alteration of certain minerals and the formation of other minerals. The distribution of sulfate varies within the weathered zone, with the top few metres having negligible amounts due to removal by rain leaching, but elevated levels may be present at the base of the root zone at around 2 to 3 metres depth, decreasing towards the base of the weathered zone, and this is identified by presence of brown staining on fissure and bedding surfaces, and presence of selenite. The weathering state is revealed by the colour changes of the iron forms present. In weathered horizons, the orange-brown colour of ferric iron predominates, whereas with depth the grey colour of ferrous iron represents less weathered lithology and indicates an increase in the presence of unreacted sulfide minerals.
Although British, European, and other standards promote good practice in carrying out investigations, potential problems are often not adequately anticipated and catered for. Historically the Building Research Establishment have provided guidance for routine UK assessment of potential ground aggressivity based upon water and acid soluble sulfate content and acidity of soil and groundwater samples. This worked well for many decades with few instances of sulfate attack on buried concrete reported. However, following investigation on several cases of sulfate attack on construction materials and disruptive ground heave during the 1980s-1990’s, it was realised that the consequences of pyrite oxidation were not being considered and had been attributed to various other assumptions. Precipitation of new minerals such as gypsum provoke possibilities of ground heave. As the process of dissolution and precipitation will not generally occur in the same location, both expansion and void creation may produce differential movements and heave causing structural damage. This necessitated revision of testing standards, and guidance advocating a staged approach based on initial review of the geological setting, followed by a planned investigation programme and detailed ground assessment. This requires an awareness of potentially aggressive material and importance of focused chemical testing. The severity of pyrite oxidation depends not only on the crystal form but also on the permeability and chemistry of the host deposits as well as the groundwater conditions. The site investigation may confirm the presence of significant quantities of pyrite, gypsum, and calcite but these values alone do not facilitate assessments of the reaction rate and significance to construction. Assessment also requires that the consequences of the construction activity and weather-related issues in the construction period and beyond are fully addressed to provide an adequate basis for the design of structures.
The consequences of pyrite reaction may become a significant hit for the construction budget and progress when unsuspected. The oxidation of pyrite bearing deposits during earthworks and construction has been observed to progress rapidly over a matter of weeks or months, producing conditions chemically aggressive to engineering materials. Therefore, to avoid or manage potential problems attributed to pyrite oxidation it is necessary to know not just that it is present, but also its distribution, its form and reactivity. Investigations and construction may overlook the potential for material deterioration, but this can be determined at a site level through observation of changes following exposure and targeted chemical testing. The distribution of sulfur compounds in soils and rocks can be highly variable so testing must ensure that sulfur-bearing horizons are not missed, and a suitable characteristic value selected for design. Material selected for laboratory testing should focus on the construction zone but also evaluate other strata that may be affected.
Knowledge of the mechanical behaviour of the host material and the changes brought about through exposure during construction may expedite management of the construction process by facilitating re-use of a favourably weathered product that would otherwise constitute an unsuitable material. Ultimately, management of material avoiding costly offsite disposal, may be achieved through informed investigation with pre-weathering of pyritic fill to mitigate the risk of heave through conversion of pyrite to selenite or by blending, encapsulation, or provision of targeted drainage and impermeable barriers.
Article provided by Mourice A. Czerewko, Associate Engineering Geologist, AECOM Ltd